Time-Division Multiplexing (TDM) is a foundational technology in telecommunications that optimizes the use of bandwidth by dividing a single physical communication channel into multiple time slots. This method allows several data streams to share the same transmission medium while keeping the integrity of each separate stream intact. By alternating segments of each data stream in rapid succession, TDM maximizes efficiency without the interference typical of other multiplexing techniques. This article explores the mechanics of TDM, its applications, advantages, and comparisons with other multiplexing methods, providing a thorough understanding of its crucial role in modern networks.
Index:
- What is Time-Division Multiplexing (TDM)?
- Components of a TDM System
- Types of TDM
- Applications of TDM in Telecommunications
- Advantages of TDM Over Other Multiplexing Techniques
- Challenges and Limitations of TDM
- References
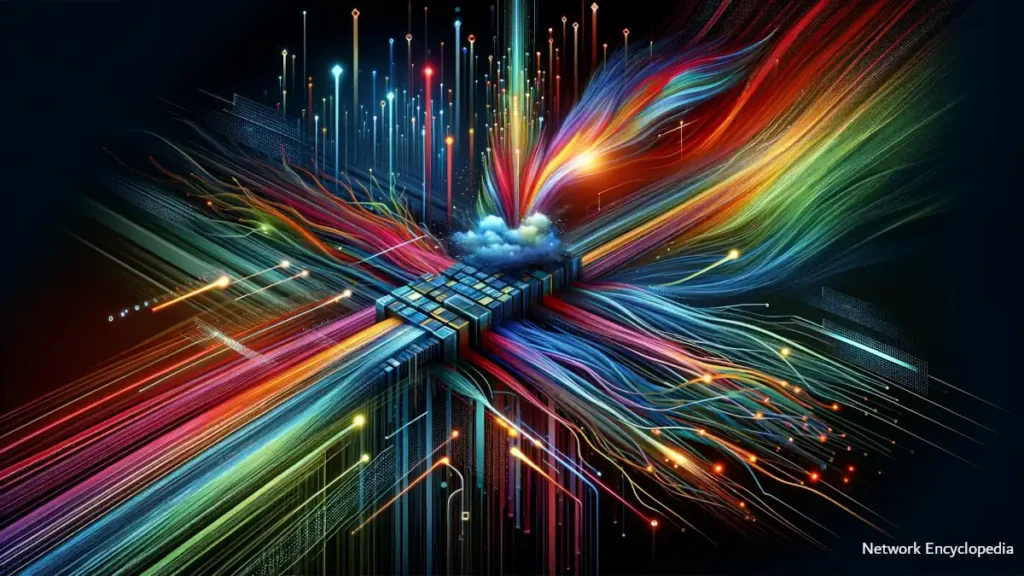
1. What is Time-Division Multiplexing (TDM)?
Time-division multiplexing is a method that allows multiple data signals to share the same transmission medium while maintaining the distinctness of each signal. This is achieved by dividing the transmission capacity of the link into time slots that are allocated to each signal. In this way, multiple signals are interleaved in time and transmitted sequentially but at such a high rate that the overall transmission appears simultaneous to the user.
1.1 How TDM works?
In time-division multiplexing (TDM), the data from different input channels is divided into fixed-length segments and then combined in round-robin fashion into a single output data stream, which can then be transmitted over a single channel transmission system and demultiplexed at the destination location. The segments can be created by the multiplexer itself or can be inherent in the input channel signals, such as fixed-length frames. For example, if input streams A, B, and C are divided into segments as shown here:
A -> A1, A2, A3,…
B -> B1, B2, B3,…
C -> C1, C2, C3,…
The output stream will look like this:
MUX(ABC) -> A1, B1, C1, A2, B2, C2, A3, B3, C3,…
One weakness in this mechanism is that if an input channel does not have anything important to carry for a time, empty segments are inserted into the output stream anyway. For example, if channel A is not transmitting data, one-third of the output channel is not being used. You can overcome this weakness by using a more sophisticated multiplexing technique called statistical multiplexing.
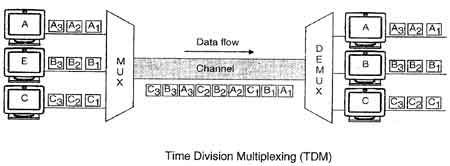
2. Components of a TDM System
Multiplexers and Demultiplexers: Functions and Mechanisms
In a TDM system, the roles of multiplexers and demultiplexers are central to its functionality. A multiplexer (MUX) for TDM combines multiple data streams into a single, high-speed output stream by assigning each low-speed stream a specific time slot within the overall signal. Each stream is thus transmitted sequentially but at a faster rate, creating the illusion that multiple communications are happening simultaneously.
At the other end of the communication line, a demultiplexer (DEMUX) performs the inverse function. It takes the high-speed incoming signal and breaks it down into its original, individual streams. This separation is based on the timing of each segment, restoring the data streams to their original rate and routing them to their respective outputs.
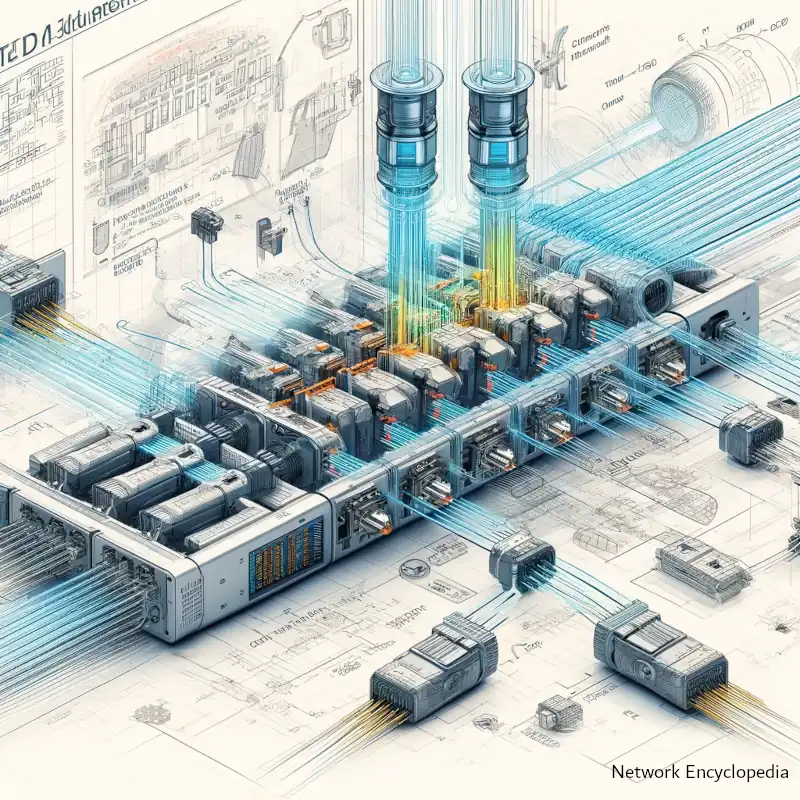
These devices are crucial because they manage the timing precision necessary for the system to function correctly. They ensure that each time slot is used to its maximum potential without overlap between the slots, which could result in data corruption.
The Role of Time Slots in TDM
Time slots are the fundamental building blocks of a TDM system. Each slot represents a specific interval during which data from a particular source is allowed to use the shared transmission medium. The division of the network’s bandwidth into discrete time slots allows multiple data streams to be interleaved and transmitted over a single physical channel.
The allocation of time slots must be highly precise, as even minor timing inaccuracies can cause data overlap, leading to errors and inefficiency. Time slots are generally of fixed duration in synchronous systems but may vary in asynchronous and statistical systems, as will be explored in the next section.
3. Types of TDM
Synchronous TDM
In Synchronous Time-Division Multiplexing, time slots are pre-assigned to sources and are fixed. This type of TDM assumes that each source always has data to send; thus, its time slot is never left empty, even if it means sending idle bits. This results in a very simple and predictable system but can lead to inefficiencies if some channels have no data to transmit while others are congested.
Asynchronous TDM
Also known as Statistical TDM, this type adjusts dynamically to the traffic load presented by the connected devices. Unlike synchronous TDM, slots are not fixed but are allocated on demand. This flexibility helps improve bandwidth utilization and efficiency because empty slots can be avoided, allowing more data-heavy streams more time as needed.
Statistical TDM
Statistical TDM is a more refined version of asynchronous TDM, where the allocation of time slots is based on statistical patterns of usage. It uses buffers to hold the data from active channels and assigns slots based on the buffer’s occupancy, giving priority to channels with more data. This method is highly efficient in environments with significant differences in channel usage, optimizing bandwidth use and reducing the likelihood of transmission delays.
4. Applications of TDM in Telecommunications
Common Uses in Industry
Time-Division Multiplexing (TDM) is widely employed across various sectors due to its efficiency and reliability. In telecommunications, TDM is fundamental for digital signal transmission where multiple signals are combined over a single transmission path. It’s used in the backbone networks that form the internet’s infrastructure, facilitating high-speed data and voice communications.
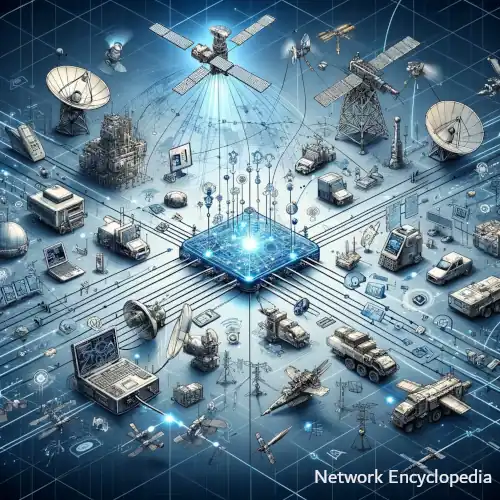
Additionally, TDM serves critical roles in mobile telecommunications, enabling efficient use of limited spectral resources among multiple users.
Case Studies: TDM in Action
1. Telephony Systems:
Traditional digital telephony systems, such as T1 and E1 lines, utilize TDM to transmit multiple voice calls over the same physical medium. This application dramatically enhances the capacity of telecommunication networks without additional cables.
2. Satellite Communications:
TDM is pivotal in managing the transmission of signals to and from satellites. By efficiently allocating time slots for multiple communications, TDM maximizes the usage of expensive and limited satellite bandwidth.
3. Military Communications:
The robust structure of TDM is ideal for military applications, where secure and reliable communication channels are crucial. TDM’s ability to separate and recombine data streams accurately ensures that communications remain secure and uninterrupted.
5. Advantages of TDM Over Other Multiplexing Techniques
Comparison with Frequency-Division Multiplexing (FDM)
While both TDM and Frequency-Division Multiplexing (FDM) are used to combine multiple data streams, they operate on fundamentally different principles. FDM allocates separate frequency bands for each data stream, allowing simultaneous transmissions over different frequencies. In contrast, TDM assigns different time slots for each stream within a single frequency band. The key advantage of TDM over FDM lies in its flexibility and efficiency in bandwidth management. Unlike FDM, TDM can dynamically allocate bandwidth based on demand, making it more adaptable to variations in data traffic.
Benefits in Terms of Bandwidth and Data Integrity
TDM’s structured approach to data transmission offers significant advantages in terms of bandwidth utilization and data integrity:
- Bandwidth Utilization: TDM maximizes the efficiency of bandwidth use by allowing the sharing of a single channel among multiple data streams. This is particularly beneficial in environments where the data load varies, as TDM can adjust the allocation of time slots to match the demand.
- Data Integrity: Since each data stream in a TDM system is transmitted in separate time slots, the risk of signal interference is minimal compared to FDM, where signals at close frequencies may interfere with each other. This separation ensures higher data integrity and reduces the likelihood of errors, making TDM a preferred choice for critical data transmission tasks.
6. Challenges and Limitations of TDM
Technical Challenges
While Time-Division Multiplexing (TDM) offers significant advantages, it also faces technical challenges that can limit its effectiveness:
- Synchronization: Maintaining precise synchronization across all transmitting and receiving devices is crucial for TDM. Any timing errors can lead to data corruption, as the demultiplexer might misalign the time slots and mix up data streams.
- Scalability: As the number of channels or the volume of data increases, TDM systems may struggle to keep up without substantial upgrades in infrastructure, making it less scalable than some newer technologies.
- Latency: In scenarios where data transmission needs are sporadic and highly variable, the fixed nature of time slots in TDM can lead to increased latency, as channels wait for their assigned slots to transmit data.
Limitations in Modern Networking Scenarios
In the context of modern digital communications, where data traffic patterns are highly dynamic and unpredictable, TDM can face limitations:
- Bandwidth Inefficiency: TDM’s fixed time slot allocation can result in unused capacity when channels have no data to send. This inefficiency is particularly problematic in networks experiencing fluctuating bandwidth demands.
- Inflexibility with High-Bandwidth Applications: High-bandwidth applications, such as video streaming and large-scale data transfers, require more flexible and dynamic bandwidth allocation than TDM can typically provide, making it less ideal in environments dominated by such applications.
- Competition with More Advanced Multiplexing Techniques: Technologies like DWDM (Dense Wavelength Division Multiplexing) and statistical multiplexing offer more granular control over bandwidth allocation and are better suited to handle the high-capacity, bursty traffic patterns of modern data networks.
7. References
To further validate and elaborate on the concepts discussed in this article, the following references provide in-depth insights and authoritative perspectives on TDM and its applications:
Books:
- Data Communications and Networking by Behrouz A. Forouzan.
- Telecommunication Switching Systems and Networks by Viswanathan Thiagarajan.
RFCs:
- RFC 4606 – “Generalized Multi-Protocol Label Switching (GMPLS) Extensions for Synchronous Optical Network (SONET) and Synchronous Digital Hierarchy (SDH) Control”
- RFC 7685 – “A One-Way Delay Metric for IP Performance Metrics (IPPM)”
Academic Papers:
- “Time-Division Multiplexing Over IP,” Journal of Network and Systems Management.
- “Challenges in the Implementation of TDM Systems,” IEEE Transactions on Communications.
Network Encyclopedia: